A primer on the origin, extraction, and emissions of everyone’s favorite fossil fuels
Three major forms of fossil energy together provide almost all the world’s energy: coal, oil (and the derived petroleum fuels), and natural gas. Moreover, humanity’s insatiable appetite for these fuels is the major driver of climate change. Therefore, it is essential to understand the fundamentals of these fuels: Where do they come from, what are their properties, and how do we extract them. Furthermore, it is generally understood that natural gas is the “cleanest” of the fossil fuels, while coal is the dirtiest. Again, we must ask why, and provide at least a basic answer. The purpose of this article is to answer (at least broadly) these questions, and to make the reader familiar with some key terms and concepts. In brief, the takeaways are:
- All fossil fuels derive from organic matter buried at great depths and processed under pressure.
- Oil and gas are produced by the same basic geologic processes, and are often present in the same wells/fields. Conventional oil/gas is formed when these hydrocarbons seep from low permeability source shales to collect in “traps,” where they can be pumped by conventional methods.
- Unconventional oil/gas extraction via “fracking” involves drilling into and breaking up source shales directly.
- Coal in the US is most commonly mined via surface mines, especially mountain top removal with valley fill, where whole mountains are blasted away to access coal seams.
- Fossil fuels are mainly a mix of molecules with carbon (C) and hydrogen (H) bonds. Burning oxidizes these molecules to water and carbon dioxide, releasing heat along the way. The more C-H bonds, vs. C-C bonds, the more energy is released per atom of C, and thus the less CO₂ per heat energy released.
- Natural gas, as CH₄, has the most C-H bonds of any fossil fuel (it is the most “reduced”), and burns the cleanest. Coal is the least reduced fossil fuel, and burns dirtiest.
Origins
All fossil fuels are formed via the burial of organic matter under inorganic sediments, leading to compression under high pressure and temperature, and the formation of carbon– and hydrogen-rich hydrocarbons. This deep burial and a lack of oxygen prevent the degradation to water and carbon dioxide that is the usual fate of dead organisms. Coal, climate enemy number one, is formed when forest matter is buried in low oxygen marshes to form peat, which then transforms into successive grades of denser and denser sedimentary coal rock. Much coal dates from the carboniferous period (359 to 299 million years ago), when the earth’s land was largely covered in marshy tropical forests. Of the three major fossil fuels, coal has the highest carbon:hydrogen ratio of the fossil fuels, stores the least energy per unit of mass, and yields the highest emissions in terms of CO₂ per kWh (see below for more on these concepts).
Oil and gas, on the other hand, are generally formed when microscopic marine life, such as algae and zooplankton, are buried in deep anoxic ocean sediments, often in a deep basin. Conventional oil and gas reserves form as follows. Organic matter in a source rock, often a shale (sedimentary rock made up of fine clay and some organic matter), is transformed by heat/pressure into oil. Between temperatures of about 65 and 150 °C, crude oil is formed (along with small amounts of gas), the so-called oil window, while at greater temperatures natural gas is formed, as shown in the following figure:
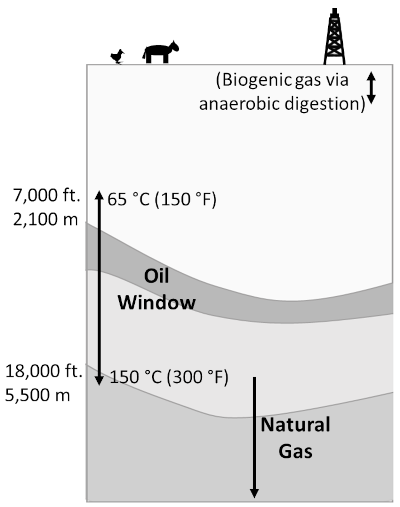
Depths and temperatures at which buried organic matter forms either oil (65–150 °C, or about 2.1–5.5 km) or natural gas (deeper/hotter). Note that anaerobic digestion by bacteria near the surface can create natural gas (i.e. methane, CH₄), as in landfills, bogs, etc. Figure by the author.
Now, oil and gas produced in shale expand, and with sufficient pressure the source rock is temporarily fractured, allowing these hydrocarbons to migrate through the subsurface, where they may encounter layers of more porous sedimentary rock.
Sedimentary rocks that are highly porous and permeability allow oil from source rocks to travel relatively freely, and these are referred to as reservoir rocks. Now, oft-times on its travels oil will encounter a trap, essentially a convexity in the reservoir rock overlain by an impermeable caprock, where these hydrocarbons can accumulate. Reservoirs may consist of either solely oil (with some dissolved natural gas, a so-called unsaturated pool); an oil reservoir overlain by natural gas — the free gas cap (a saturated pool); or a pure gas reservoir. Water, usually highly saline brine, is present at depth and occurs at the base of all reservoirs (water is much denser than oil or gas). This general process, and the possible reservoir types are illustrated as follows:
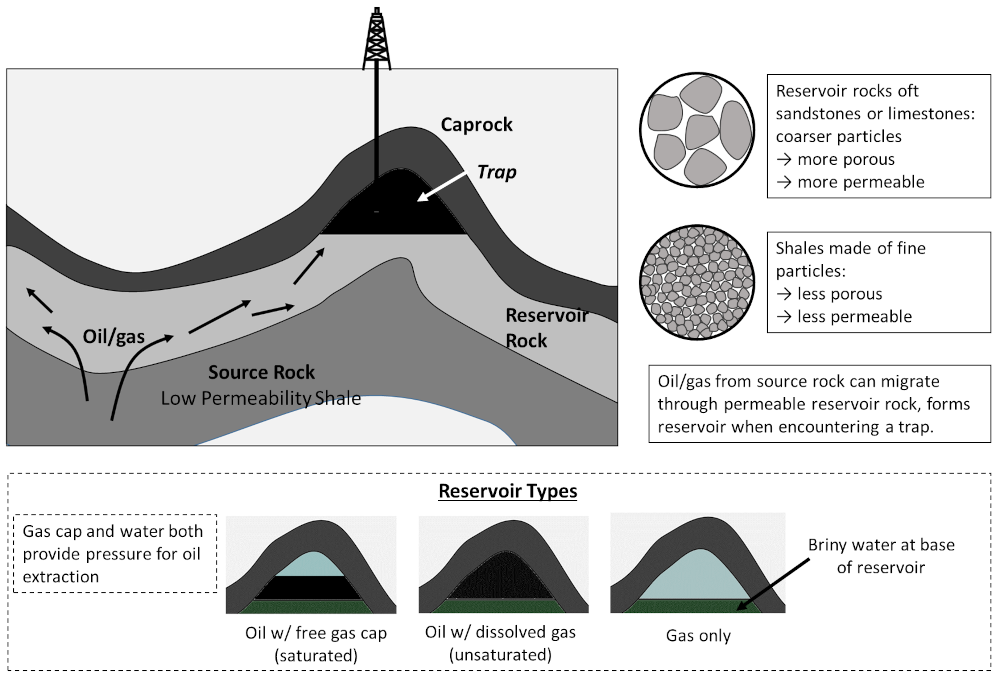
Schematic for the formation of conventional oil and gas reservoirs, whereby oil/gas from low permeability source rocks enters high permeability reservoir rocks, where it may migrate into a trap overlain by an impermeable caprock. Reservoirs so formed may consist of gas only, a gas layer above an oil layer (saturated), or gas mixed in with oil (unsaturated). Figure by the author.
Composition and Basic Properties of Crude Oil and Natural Gas
Crude oils consist of a mix of straight hydrocarbon chains (alkanes), closed hydrocarbon rings (naphthenes), and aromatic hydrocarbon rings. Oils consisting primarily of shorter hydrocarbon chains are light, while longer, dense hydrocarbon mixes are heavy. Depending on its sulfur content, oil is referred to as sweet (low sulfur) or sour (high sulfur), with light, sweet oils the most valuable. All crude oil begins light, but when it seeps to the surface (or near surface), the lighter fractions may be lost to evaporation and water flows, or they may be degraded by bacteria, yielding lower quality heavy oils. The Alberta tar sands are an example of a highly degraded, heavy bituminous oil.
Looking just as hydrocarbon chains, i.e. alkanes, we have the following sequence of basic alkanes, from methane through pentane:
The pattern continues as expected, and our general formula for an n-alkane (n being the number of carbon atoms) is Cₙ H₂ₙ₊₂. Again, the longer the chain, the heavier the hydrocarbon and the denser the fuel.
At the refinery, crude oil is separated into fractions via distillation, such that the lightest fractions (composed of the smallest molecules) are gaseous, and include liquid petroleum gas (LPG) and propane, followed by gasoline. Kerosene, which is the basis for jet fuel, is of medium density, while heavier fractions make up diesel and heavy fuel oils, with bitumen/asphalt the densest and heaviest of all. Gasoline is generally the most valuable fraction, and therefore some heavier hydrocarbons are processed to gasoline via cracking.
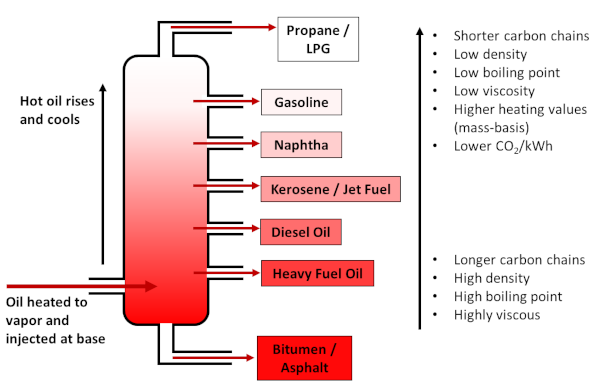
Distillation of crude oil into various fractions by density/boiling point. Lighter fractions (i.e. shorter hydrocarbon chains) store more energy per carbon atom and yield lower CO2 emissions. Figure by the author.
Natural gas from the field varies in its exact composition. It is always mostly methane, but small amounts of ethane and nitrogen gas can be present, as may trace amounts of butane, propane, and hydrogen sulfide (a gas with significant sulfur content is a sour gas). Natural gas as delivered to customers is nearly pure methane.
Unconventional Oil and Gas
Conventional gas and oil reservoirs, as just discussed, are formed when hydrocarbons from low permeability source rocks, typically clay shales with high organic content, seep into higher permeability reservoir — sandstone or carbonate — rocks. Unconventional oil and gas come from tight formations, either sandstones or carbonate formations of low permeability, or the low permeability source shales themselves. By 2021, the US EIA has estimated that 79% of US natural gas and 65% of US oil came from unconventional sources.
Extraction from tight formations, especially shale, did not become economical until the advent of horizontal drilling techniques and hydraulic fracturing. With horizontal drilling, a single well-bore is drilled horizontally through a formation. Then, fracking fluid, a mixture of mainly water and proppants (e.g. sand) is injected under high pressure, creating fractures in the shale and/or opening existing fractures. When the injection pressure is relieved, proppants hold the fractures open. This process is illustrated in the following figure, along with some of the potential routes for methane leakage and effects on water supplies.
Fracking for unconventional oil and gas has been extremely controversial, as the reader is likely aware. One of the principal environmental concerns is fugitive methane emissions that result from the extraction process (as well as during distribution, etc.), which at least partially offset the advantage of gas over coal for electricity generation, as methane is not only a fossil fuel, but a greenhouse gas in its own right. Other concerns include effects on local water, increased seismic activity from what are, essentially, controlled earthquakes, as well as habitat destruction and landscape fragmentation from extensive exploration of oil and gas fields. An example of such fragmentation follows
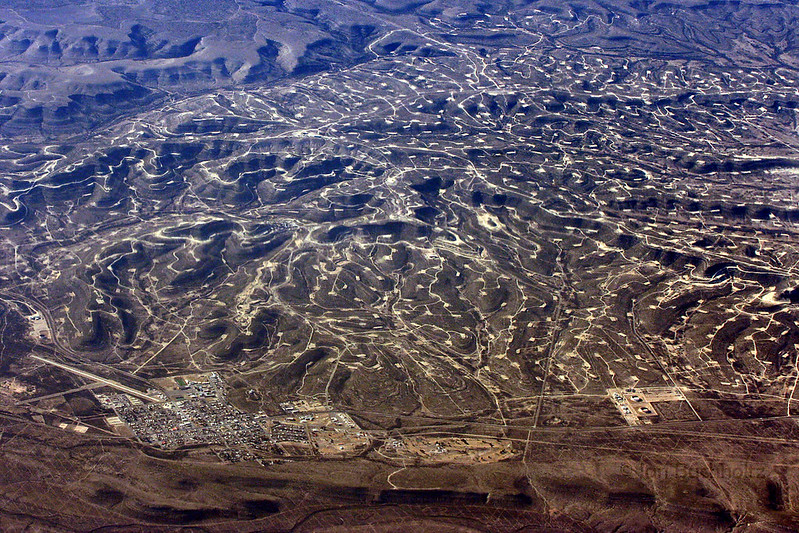
An example of landscape fragmentation due to extensive oil and gas exploration in the Yates field in Texas. Figure by Jan Buchholtz (link).
Coal Extraction
Coal was traditionally extracted from underground mines, but since around 1975 coal production has been increasingly via surface mining, with about two-thirds of coal in central Appalachia extracted from surface mines in recent years. A common method is mountaintop mining with valley fill (MTM/VF), whereby whole mountaintops are cleared of forest, blasted away, and mined, while nearby valleys and streams are buried with the waste rock. Valley fills can stretch for over a mile, and may be hundreds of meters deep. Such mining is a major driver of land-use change in central Appalachia, and 5% of southern West Virginia had been converted to mine in 2005 [1].
The consequences of MTM/VF are many. Most obviously, large tracts of deciduous forest are lost directly to mining, while the forests that remain are highly fragmented. The headwater streams buried by valley fills are ecologically sensitive and hydrologically important (as the primary water sources for most rivers), and their burial and contamination affects downstream river networks [1]. Such large-scale altering of the landscape topology also fundamentally alters the regional hydrology, and exposure to contaminated water and airborne dust in mined areas may negatively affect human health as well.
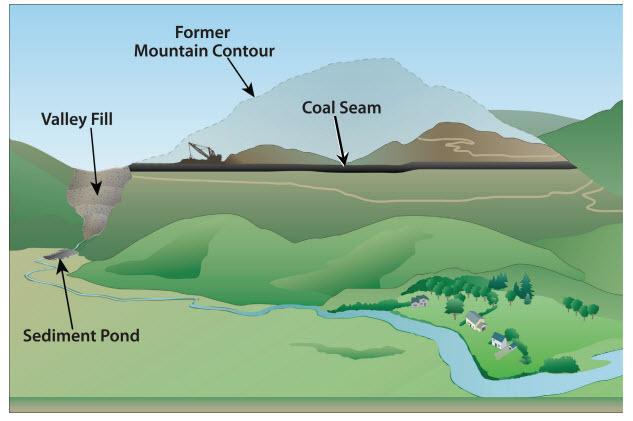
Schematic for coal mining via mountain top removal with valley fill. Figure by US EPA; US Government work.
Carbon Intensity of Coal, Oil, and Gas
To understand why fossil fuels vary in carbon intensity (that is, the amount of CO₂ released per unit of energy generated), we need to take a bit of a detour into chemistry, energy storage, and the notions of respiration and oxidation. The cyclical conversion of energy, H₂O, and CO₂ to energy-storing carbohydrates and O₂, and visa versa, is the energetic basis for almost all life on earth, with photosynthesis representing the energy storage pathway, and respiration the energy-releasing pathway. The basic reaction for photosynthesis is
6CO₂ + 6H₂O → C₆H₁₂O₆ + 6O₂.
The product on the right hand side of the equation is glucose, which can be converted to a variety of other biological molecules that act to store energy, such as lipids (long-chain hydrocarbons), or have a structural role, such as cellulose (which also stores energy). Oil and gas are the result of anoxic breakdown of energy-storing organic molecules, as we have already discussed, yielding hydrocarbons with just C and H, and a mix of C-C and C-H bonds.
Now, when the C-O bonds of CO₂ are converted to C-C and C-H bonds, we say that the carbon has been reduced, and C-H bonds are more reduced than C-C bonds. Reduced bonds store energy. Thus, the more highly reduced the carbon in a molecule, the more energy it stores per carbon atom. It follows that methane, with a 1:4 C:H ratio stores more energy per carbon atom than, for example, pentane, with a 1:2.4 C:H ratio.
Oxidation, which is essentially synonymous with respiration, is the process of oxidizing reduced bonds to release energy. Hydrocarbons interact with O₂ to yield H₂O, CO₂, and heat, and our general formula for oxidizing (or combusting) a hydrocarbon with the formula CₓHᵥ is given as:
CₓHᵥ + (x + v/4)O₂ → xCO₂ + (v/2)H₂O + Heat
So, for example, if we combust methane, we get
CH₄ + 2O₂ → CO₂ + 2H₂O + Heat
Combusting pentane gives us the reaction:
C₅H₁₂ + 8O₂ → 5CO₂ + 6H₂O + Heat
This second reaction yields less heat per carbon molecule, resulting in higher CO₂ emissions for the energy produced. From the properties of different bonds, it is possible to calculate the energy released in each reaction (I will spare you, gentle reader, the details here), and determine an emissions factor that gives kgCO₂ released per kWh of energy generated, for each alkane. The emissions factor is about 25% lower for natural gas (methane) than most other petroleum fractions.
Coal, unlike natural gas and petroleum-based fuels, is highly heterogeneous, and is composed of a mixture of non-combustible minerals (ash), water, and complex carbon chains with hydrogen and oxygen. On the whole coal is far less reduced than either oil or gas, and thus has a much higher emissions factor. Coal also tends to contain 1–2% sulfur, to the detriment of local air quality. Coals come in different grades, and those containing more hydrogen by mass emit somewhat less CO₂ per kWh, but all have a very high CO₂/kWh ratio. The following figure illustrates the emissions factors for natural gas (methane), various petroleum fractions, and coal grades:
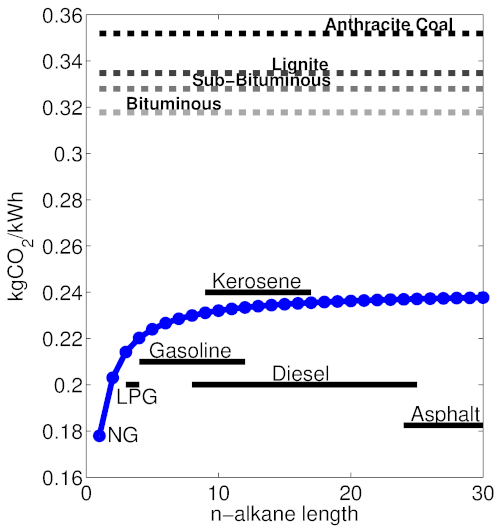
Theoretical emissions factors for alkanes of various lengths in terms of kgCO₂ released per kWh of heat energy. This metric for different ranks of coal is also provided, based on [2]. Approximate chain-lengths for common petroleum fuels are demonstrated. Figure by the author; see [3] for details on the theoretical calculations.
Summary
To sum up, different sources of organic matter which store energy as (partially) reduced carbon can be buried deep underground by different geologic processes, where they may be transformed into either coal, oil, or natural gas (methane). Oil and natural gas are created by the same basic processes, and are typically extracted in the same fields and even wells.
Oil and gas form in tight low permeability shales, from which they may migrate to collect in conventional reservoirs. Unconventional oil/gas extraction involves drilling into the shales themselves and increasing permeablity through hydraulic fracturing. Most oil and gas produced in the US is now unconventional. Most US coal is extracted in large surface mines that can be highly destructive to local landscapes.
Crude oil is refined into different fractions, based on hydrocarbon chain length and density. Heavy fuel oils and diesel are examples of heavy fractions, while gasoline and propane are light fractions.
Natural gas, different petroleum fractions, and coal, all have different C:H ratios (a lower C:H ratio implies more reduced carbon). In general, the more reduced the carbon in the fuel, the more energy is stored, and the less CO₂ per kWh of energy released. Methane is the most reduced fossil fuel (lowest C:H ratio) and has the lowest emissions, while coal is the least reduced and has the highest emissions.
For far more technical detail on all this, you may consult A Fair Share: Doing the Math on Individual Consumption and Global Warming, by the author.
(See also medium.com version)
References
[1] Bernhardt, E. S., Lutz, B. D., King, R. S., Fay, J. P., Carter, C. E., Helton, A. M., … \& Amos, J. (2012). How many mountains can we mine? Assessing the regional degradation of central Appalachian rivers by surface coal mining. Environmental science & technology, 46(15), 8115–8122.
[2] Hong, B. D., & Slatick, E. R. (1994). Energy Information Administration, Quarterly Coal Report, January-April 1994, DOE/EIA-0121(94/Q1) (Washington, DC, August 1994), pp. 1–8. https://www.eia.gov/coal/production/quarterly/co2_article/co2.html#N_5_
[3] Eikenberry, S. E. (2018). A fair share: Doing the math on individual consumption and global warming. 1st ed. Ingram Publishing; 510 p.
4462ww